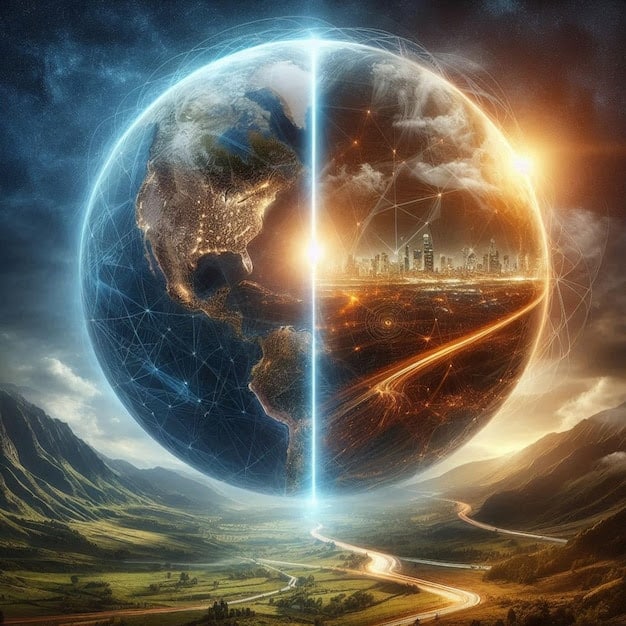
The laws of mass and energy conservation apply to planet Earth as to any physical system. For the Earth, almost all energy exchanges with its cosmic environment are made up of radiative exchanges: radiation received from the Sun (in the visible light, near-infrared and ultraviolet wavelength ranges) and heat radiation emitted from the Earth (in the middle infrared). The radiative balance of the Earth can be understood as a global balance of energy, close to zero since the planet is not very far from equilibrium. The balance of energy gains and losses is written as follows:
“Net global balance = absorbed solar radiation + internal heat production – thermal infrared radiation emitted to space”
It should also be noted that the heat production from natural radioactivity inside the Earth is very low (about 0.1 watt per square meter) compared to the radiative flux absorbed or emitted by the planet (239 W • m–2). The production of non-renewable energy by human activities is negligible, even if it produces heat islands in large cities.
The elements of the global radiative balance of the Earth
It is more informative to examine the elements of this global balance, that is to study in detail the balance of radiation exchanges of different types between the planet, the Sun and space according to place and time. After all, you can balance your financial assets year over year to see if you have more or less savings or debt, but it’s still important to know where your money comes from and what expenses you’ve spent it on.
It should be noted that the following explanations relate to the radiative balance at the “top” of the atmosphere, which can be located, by convention, at 30 km, since very little infrared or solar radiation is emitted or reflected above this altitude. The atmosphere extends well over 30 km, with no net limit, but for the lifetime of satellites this limit is approximately between 500 and 800 km.
The balance of solar radiation
Solar radiation is close to 1,365 W • m–2 on a plane facing the Sun at the average distance between the Sun and the Earth (1 astronomical unit or AU). But the earth is round and you have to divide this value by four
This value must be divided by two first time, because only half of the earth is illuminated by the sun, and a second time by two because of the inclination of the sun’s rays with respect to the earth’s surface.
To obtain the mean incident flux at the top of the atmosphere near 341 W • m–2.
Also, because the Earth’s orbit is slightly elliptical, the solar flux at the top of the atmosphere is greater when our planet is closer to the Sun, which happens in January. This flux is weaker in July when the Earth is furthest from the Sun.
Of course, the inclination of the axis of rotation of the Earth means that the solar flux received in the winter hemisphere is less than that received in the summer hemisphere, for each of these two cases.
On average, the planet reflects and emits about 30% of the solar flux incident to space. The remaining 70% of solar flux, or 239 W • m–2 absorbed (239.4 W • m–2 according to Trenberth et al., 2009
Trenberth, Kevin E. et al., 2009: Earth’s global energy budget. Bulletin of the American Meteorological Society, 90 (3), 311-323.
), are converted into heat on the planet’s surface and in the atmosphere. Specialists speak of the overall balance of “short wave” radiation because the main wavelengths of solar radiation range from 0.2 micrometers (μm), in the ultraviolet, to the range between 0.4 and 0.9 μm for visible light, and up to 4 μm in the near infrared.
Short wave balance = incident solar radiation – reflected solar radiation
The balance of thermal infrared radiation
To maintain a rough balance and a global radiative balance close to zero, the planet must “get rid” of this heat from solar origin that is constantly arriving. The only possibility for the Earth is to radiate into space. This medium infrared radiation, also called thermal, is mainly emitted at wavelengths between 3 and 60 μm. The overall “short wave” balance of +239 W • m–2 corresponds to a global “long wave” balance close to –239 W • m–2.
Long wave (LW) balance = infrared thermal radiation emitted to space.Based on Trenberth et al. (2009). Graphic adaptation: Elsa Godet. Excerpt from: Jeandel C. and R. Mosseri (ed.), Le Climat à découvert, Paris, CNRS Éditions, 2011, p. 47.
The arrows represent energy flows in proportion to their intensities. In the long-wave field, radiation emitted from the surface (396 W • m–2) is partly transmitted directly to the top of the atmosphere without being absorbed in transit (40 W • m–2). The rest is absorbed by the atmosphere and clouds. Part of the “long wave” radiation is thus emitted into space by the atmosphere (169 W • m–2) and by clouds (30 W • m–2).
This “long wave” balance practically balances the “short wave” radiation balance, which results from the difference between what is received from the Sun (341 W • m–2) and what is reflected (102 W • m–2). In the end, the balance between these two balances can be written: 40+169+30=341-102=239.
The deviations to the equilibrium of the radiative balance of the Earth
At present, the planet is not in equilibrium even if it is averaging over the year and the overall radiative balance is almost certainly not zero. The main cause of this imbalance is not the variation of the solar constant. This varies by only 0.1% with the 11-year solar activity cycle, a fluctuation of 0.24 W • m–2 when considering the solar radiation converted into heat (0.1% of 239 W • m–2).
Sometimes, as a result of powerful volcanic eruptions, the planet reflects a little more solar radiation and absorbs a little less. But above all, over the past few decades, human activities have changed the composition of the atmosphere, particularly with increasing emissions of carbon dioxide (CO2). These emissions cause an increase in the absorption of infrared radiation into the atmosphere, reinforcing the greenhouse effect.
As the continental surfaces and ocean surface layers warm, infrared heat radiation escaping into space is decreasing. It is estimated that this decrease should be close to 1 W • m–2 even if it is still difficult to measure with confidence.
The overall “long wave” balance increases from –239.4 W • m–2 to –238.5 W • m–2 and the overall radiative balance increases by about 1 W • m–2, according to Trenberth et al. 2009.
Only when the planet reaches a new equilibrium will the global radiative balance be close to zero again. This new equilibrium can only be achieved if the composition of the atmosphere stabilizes and, as it contains more CO2, the average temperature on the surface of the Earth will therefore be higher.
Structure of the radiative balance of the Earth in space and time
Let’s take a closer look at the elements that make up the overall radiative balance of the Earth, starting with the top of the atmosphere. This is particularly important because measurements – mainly from space and taken by different artificial satellites – are carried out at different points in the world and at different times, in the cycle of seasons and the day-night cycle.
The solar balance (or «short wave» balance) is necessarily zero at night (which includes the polar night for months): nothing happens and nothing is reflected. During the day, especially in the tropics and in temperate or subpolar areas in summer, this balance is positive and can be strong where there are no clouds or ice to reflect the Sun. Measurements indicate that clouds account for half of the 102 W • m–2 solar reflected into space.
On the other hand, the “long wave” balance remains negative at night as well as during the day, in winter and summer. Temperatures on the Earth’s surface and in the atmosphere never drop to absolute zero (-273°C) due to the retention of heat below the surface and the transport of heat by movements of the atmosphere and oceans. Thermal infrared radiation towards space is particularly strong over deserts in summer.
But clouds – especially high and cold clouds – also block infrared radiation to space. In tropical regions, thermal infrared radiation is strong where there are no clouds, or only low clouds, but it is very weak above the thick and high clouds of the intertropical front. The measurements indicate that, overall, without clouds, the “long wave” balance would be more negative than 30 W • m–2 and, because of the decrease in reflected solar radiation (50 W • m–2), the planetary radiative balance would then be +20 W • m–2 (the difference between the gain in solar radiation of 50 W • m–2 and the loss in infrared of 30 W • m–2). Without clouds, the planet would be at a much higher temperature.
The net radiative balance varies greatly with latitudes and seasons: it is positive in summer and negative in winter. Although it is not very cold in France in winter, when the regional net radiative balance approaches –100 W • m–2, it is because atmospheric and oceanic movements bring heat from more southern regions where the net radiative balance is positive. And in summer, our mid-latitudes export more heat to the Arctic than they do in winter.
All of the above refers to the elements of the radiative balance at the «top» of the atmosphere, therefore to the exchanges between the Sun, the Earth and space. We can and must also study the elements of the radiative balance on the surface of the Earth. But be careful! In addition to radiative exchanges, the surface also exchanges heat with the atmosphere through other mechanisms.
The surface receives descending heat radiation from the atmosphere (an essential aspect of the natural greenhouse effect) while the «long wave» radiation coming from cosmic space is practically nil. The net radiative balance at the surface, on average, on the globe and over 24 h, is generally positive and, in the absence of an imbalance in the planet’s energy balance, it is exactly balanced by non-radiative heat losses to the atmosphere (Sensitive heat flux and latent heat flux).
Under current conditions, the energy balance on the Earth’s surface also increases by about 1 W • m–2, according to Trenberth et al., 2009.
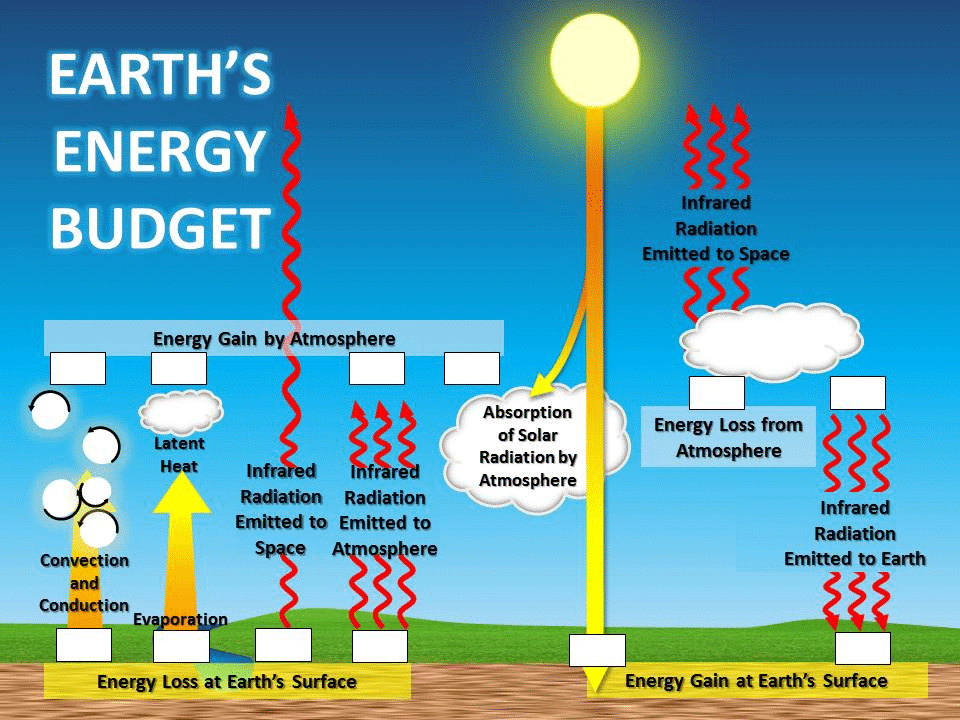
The symbols have the following meanings:
Top of the atmosphere (TOA):
SW (Short Wave): solar radiation (“short wave”);
LW (Long Wave): thermal infrared radiation (“long waves”);
LE (Latent Evapotranspiration): latent heat flux from the surface to the atmosphere;
H (Heat): Sensitive heat flux.
The planetary averages of the elements of the radiative balance of the Earth at the top of the atmosphere (TOA) are, from left to right: the incident solar radiant flux, the reflected solar flux and the emitted long wave thermal flux. On the surface of the Earth are represented, from left to right: the solar flux descending, the solar flux ascending (reflected), the non-radiative flux of latent heat (LE) and sensitive heat (H), the long-wave flux descending, and the emission of the long-wave flux ascending. All these flows are in the range of 10 to 350 W • m-2 (except for the solar flux at night, zero). The mean flux from the interior of the Earth to its surface (about 0.1 W • m-2) is not shown.
Source : Kandel, R. et Viollier, M., 2010 : Observation of the Earth’s Radiation Budget from Space. Comptes Rendus Géosciences, 342, 286-300.
https://www.sciencedirect.com/science/article/pii/S1631071310000167
https://cleanet.org/details/files/28510.html
https://journals.ametsoc.org/view/journals/bams/90/3/2008bams2634_1.xml